
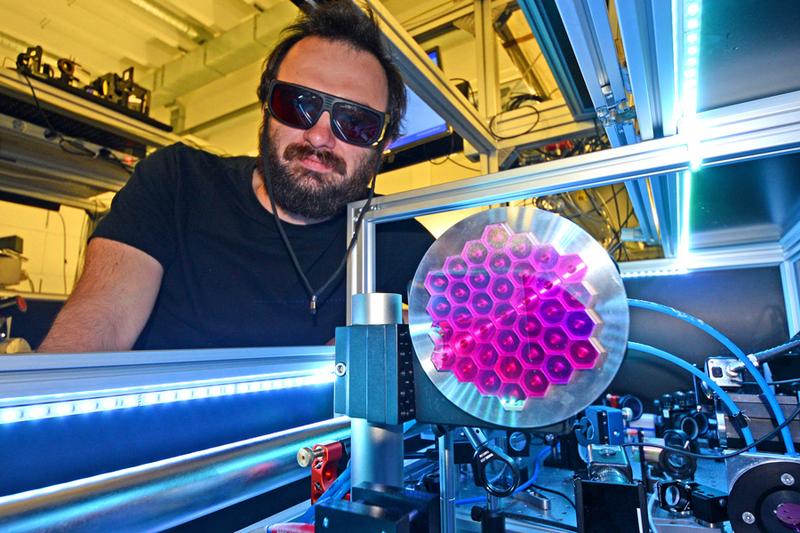
The ATLAS Lasersystem based in the Laboratory for Extreme Photonics of the Ludwig-Maximilians University Munich, serves as a light source for the new brilliant X-ray radiation.
Thorsten Naeser
For over a century, medical imaging has made use of X-rays produced in a specialized type of vacuum tube. The major disadvantage of this method lies in the poor quality of the emitted radiation. The source emits radiation from a large spot into all directions and over a broad energy range.
These features are responsible for the relatively modest resolution attainable with this mode of imaging. X-rays generated in synchrotrons provide much higher resolution, but their dimensions and cost preclude their routine use in clinical settings.
However, an alternative approach is now available, for two laser pulses can generate X-rays of similar quality to synchrotron radiation in devices with a far smaller footprint: One pulse accelerates electrons to very high energy and the other forces them into an undulating motion. Under these conditions, electrons emit X-radiation that is both highly energetic (‚hard‘) and highly intense, and is therefore ideal for probing the microscopic structure of matter.
Now, physicists based at the Laboratory for Attosecond Physics (LAP) at LMU Munich and the Max Planck Institute for Quantum Optics (MPQ) have developed such a laser-driven X-ray source for the first time. With the aid of two laser pulses, the researchers have generated ultrashort bursts of X-rays with defined wavelengths tailored for different applications.
The new source can image structures of varying composition with a resolution of less than 10 micrometers. This breakthrough opens up a range of promising perspectives in materials science, biology and – in particular – medicine.
Imaging of microscopic structures in any sample of matter requires the use of a very brilliant beam of light with a very short wavelength. Brilliant radiation is able to concentrate a maximum amount of light quanta or photons of a single defined wavelength within the smallest area and shortest duration. Hard X-radiation is therefore ideal for this purpose, because it penetrates matter and exhibits wavelengths of a few hundredths of a nanometer (few-hundredths of a billionth of a meter, 10^-11 m).
Unfortunately, the only sources of high-intensity beams of hard X-rays so far available are particle accelerators, which are typically huge and highly expensive. But there is, in principle, a far more economical and compact way of doing the job – with optical light.
A team at the Laboratory for Attosecond Physics, which is run jointly by LMU and the MPQ, has now taken an important step towards realizing this goal. Led by Prof. Stefan Karsch and Dr. Laszlo Veisz, the scientists have succeeded in generating bright beams of hard X-radiation by purely optical means. Moreover, the wavelength of the emitted radiation can be readily adjusted to cater for different applications.
The physicists focused a laser pulse, lasting 25 femtoseconds and packing 60 terawatts (6×10^13 Watts) of power, onto a fine jet of hydrogen gas. Note here that the output of a nuclear power station – 1500 MW (1.5×10^9 Watts) – is very modest by comparison, but each pulse only lasts for 25 millionths of a billionth of a second. The strong electric field associated with each pulse knocks negatively charged electrons out of the gas, giving rise to a cloud of ionized particles, or ‘plasma’.
The wavefront courses through the plasma like a snow-plow, sweeping the electrons aside and leaving behind the positively charged atoms (which are much heavier). The separation of oppositely charged particles generates very strong electrical fields, which cause the displaced electrons to whiplash back and forth.
This in turn creates a wave-like pattern within the plasma, which propagates in the wake of the laser pulse, rather like the trailing wave caused by the keel of a speedboat racing on a lake. A fraction of the free electrons are caught up in this wave and can effectively ride on it like a surfer, directly behind the advancing laser pulse. Indeed, in this ‘wakefield’, the surfing electrons can be rapidly accelerated to velocities very near the speed of light.
When the electrons have reached their maximal speed they are allowed to collide head-on with a counter-propagating laser pulse, creating a so-called optical undulator whose oscillating electric field causes the free electrons to oscillate along a direction perpendicular to their direction of propagation. Highly energetic electrons that are forced to oscillate in this way emit radiation in the form of X-ray photons with wavelengths as short as 0.03 nm.
In addition, in these experiments, the higher harmonics (waves whose frequency is an integer multiple of the fundamental frequency) entrained on the electron motions by the light field could be detected directly in the X-ray spectrum – a feat that has been attempted many times on conventional particle accelerators without success.
One of the great advantages of the new system in comparison with conventional X-ray sources is that the wavelength of the emitted light can be tuned over a wide range. This ability to alter the wavelength allows radiologists to analyze different types of tissue, for instance. By fine-tuning the incident beam, one can gain the maximum information about the sample one wishes to characterize.
Not only is the laser-driven radiation tunable and extremely bright, it is produced in pulsed form. Each 25-fs laser pulse gives rise to X-ray flashes of a few fs duration. This makes it useful for applications such as time-resolved spectroscopy, which is used to investigate ultrafast processes at the level of atoms and electrons.
The intensity of the pulses (i.e. the number of photons per pulse) generated by the new source is not yet high enough for this task, but the researchers hope to overcome this obstacle with the aid of the facilities at the new Centre for Advanced Laser Applications (CALA), now being built on the Garching Campus.
The new optically generated radiation can also be combined with phase-contrast X-ray tomography, an imaging procedure that is being refined by Prof. Franz Pfeiffer of the Technical University of Munich (TUM). This technique extracts information from the light that is scattered (rather than that absorbed) by an object.
“Using this method, we can already image structures as small as 10 micrometers in diameter in opaque materials,” Stefan Karsch explains. “With our new X-ray source, we will be able to obtain even more detailed information from living tissues and other materials,” he adds.
Original publication:
K. Khrennikov, J. Wenz, A. Buck, J. Xu, M. Heigoldt, L. Veisz, and S. Karsch
Tunable All-Optical Quasimonochromatic Thomson X-Ray Source in the Nonlinear Regime
Physical Review Letters 114, 195003 (2015), 14th May 2015
Contact:
Prof. Dr. Stefan Karsch
Faculty of Physics, LMU Munich
Am Coulombwall 1
85748 Garching
Phone: +49 (0) 32905-242
Email: stefan.karsch@mpq.mpg.de
http://www.attoworld.de – Homepage of the Laboratory for Attosecond Physics (LAP)
http://www.lex-photonics.de – Homepage of the Laboratory for Extreme Photonics (LEX)